30-second summary
Electric Car Battery
An electric car battery (known as the electric vehicle battery – EV battery) is a rechargeable battery used to power the electric motors of a battery electric vehicle (BEV) or hybrid electric vehicle (HEV). Electric car batteries differ from starting, lighting, and ignition (SLI) batteries as they are designed to give power over sustained periods of time and are deep-cycle batteries.
Batteries for electric vehicles are characterized by their relatively high power-to-weight ratio, specific energy, and energy density; smaller, lighter batteries are desirable because they reduce the vehicle’s weight and improve its performance.
Today, the most common battery type in modern electric vehicles are lithium-ion and lithium polymer because of their high energy density compared to their weight.
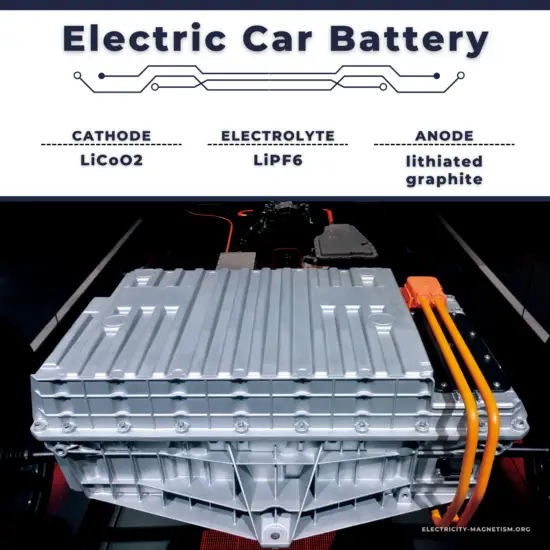
Electric Car Battery
Electric vehicles have reemerged as a viable alternative means of transportation, driven by energy security concerns, pressures to mitigate climate change, and soaring energy demand. The battery component will play a key role in the adoption of these vehicles as it defines their cost, range, and safety. An electric car battery (known as the electric vehicle battery – EV battery) is a rechargeable battery used to power the electric motors of a battery electric vehicle (BEV) or hybrid electric vehicle (HEV). Electric car batteries differ from starting, lighting, and ignition (SLI) batteries as they are designed to give power over sustained periods of time and are deep-cycle batteries. Batteries for electric vehicles are characterized by their relatively high power-to-weight ratio, specific energy, and energy density; smaller, lighter batteries are desirable because they reduce the vehicle’s weight and improve its performance.
Previous battery technologies failed to provide the required specifications, particularly in terms of driving range. However, recent developments in lithium-ion battery technology have eliminated these limitations. Today, the most common battery type in modern electric vehicles are lithium-ion and lithium polymer because of their high energy density compared to their weight. Advances in lithium-ion battery technology are creating possibilities for electric vehicles to compete with their gasoline counterparts for the first time. However, many challenges remain, the most important of which is cost.
Cell → Module → Pack
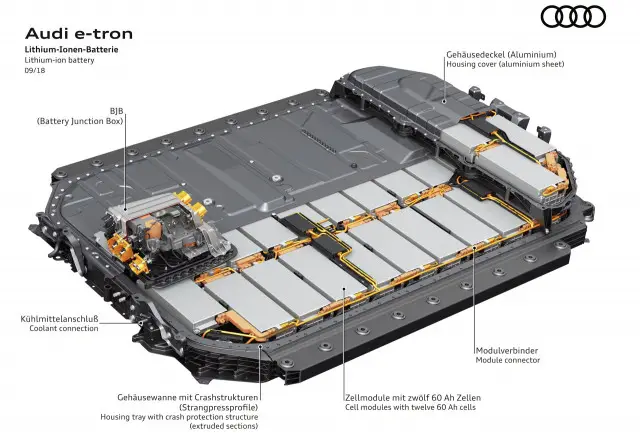
The composition of an EV battery might vary slightly depending on the types of electric vehicles, but generally, EV batteries are composed of
- Electrochemical Cells. An electric cell is essentially a source of DC electrical energy. It converts stored chemical energy into electrical energy through an electrochemical process.
- Battery Modules. A battery module is an assembly of battery cells, which is put into the frame by combining a fixed number of cells to protect the cells from vibration, heat, or external hazards. A battery module will always incorporate many discrete cells connected in series and parallel to achieve the module’s total voltage and current requirements.
- Battery Pack. The final shape of an electric vehicle battery installed to an electric vehicle. The collection of data from the pack sensors and activation of the pack relays are accomplished by the pack’s battery monitoring unit (BMU) or the battery management system (BMS).
An electric car battery is composed of many electrochemical cells. The actual battery cells can have different chemistry, physical shapes, and sizes, as preferred by various pack manufacturers. To operate an electric vehicle, an enormous amount of power a thousand times stronger than that of a smartphone is required. That is why EVs need dozens of battery cells up to as many as thousands. The large stack of cells is typically grouped into smaller stacks called modules. Several of these modules are placed into a single pack. The cells are welded within each module to complete the electrical path for current flow. Modules can also incorporate cooling mechanisms, temperature monitors, and other devices.
Lithium-ion Battery
A lithium-ion battery, also known as the Li-ion battery, is a type of secondary (rechargeable) battery composed of cells in which lithium ions move from the anode through an electrolyte to the cathode during discharge and back when charging.
The cathode is made of a composite material (an intercalated lithium compound) and defines the name of the Li-ion battery cell. The anode is usually made out of porous lithiated graphite. The electrolyte can be liquid, polymer, or solid. The separator is porous to enable the transport of lithium ions and prevents the cell from short-circuiting and thermal runaway.
Chemistry, performance, cost, and safety characteristics vary across types of lithium-ion batteries. Handheld electronics mostly use lithium polymer batteries (with a polymer gel as electrolyte), a lithium cobalt oxide (LiCoO2) cathode material, and a graphite anode, which offer high energy density.
Li-ion batteries, in general, have a high energy density, no memory effect, and low self-discharge. One of the most common types of cells is 18650 battery, which is used in many laptop computer batteries, cordless power tools, certain electric cars, electric kick scooters, most e-bikes, portable power banks, and LED flashlights. The nominal voltage is 3.7 V.
Note that non-rechargeable primary lithium batteries (like lithium button cells CR2032 3V) must be distinguished from secondary lithium-ion or lithium-polymer, which are rechargeable batteries. Primary lithium batteries contain metallic lithium, which lithium-ion batteries do not.
Types of Lithium-ion Batteries for Car Batteries
The cathode is made of a composite material (an intercalated lithium compound) and defines the name of the Li-ion battery cell. There are two kinds of electrodes: intercalation and conversion electrodes. The intercalation electrodes are materials that function as host materials where lithium ions can intercalate. A typical example is LiCoO2 and its derivatives. Conversion-type cathode materials are some of the key candidates for the next generation of rechargeable Li and Li-ion batteries.
One of the most common lithium batteries is:
- Lithium Cobalt Oxide (LiCoO2). LiCoO2 is the most commonly used cathode material. LiCoO2 batteries have very stable capacities, although their capacities are lower than those based on nickel-cobalt-aluminum (NCA) oxides. However, cobalt is relatively expensive compared to other transition metals, such as manganese and iron, despite the attractive electrical properties of LiCoO2 cathodes. Currently, we can find this type of battery in mobile phones, tablets, laptops, and cameras.
- Lithium Manganese Oxide (LiMn2O4). LiMn2O4 is a promising cathode material with a cubic spinel structure. LiMn2O4 is one of the most studied manganese oxide-based cathodes because it contains inexpensive materials. A further advantage of this battery is enhanced safety and high thermal stability, but the cycle and calendar life is limited. This type of battery is found in power tools, medical devices, and powertrains.
- Lithium Nickel Manganese Cobalt Oxide (LiNiMnCoO2) – NMC. Nickel manganese cobalt (NMC) batteries contain a cathode made of a combination of nickel, manganese, and cobalt. NMC is one of the most successful cathode combinations in Li-ion systems. It can be tailored to serve as energy cells or power cells like Li-manganese. NMC batteries are used for power tools, e-bikes, and other electric powertrains.
- Lithium Iron Phosphate (LiFePO4) – LFP. LiFePO4 is one of the most recent cathode materials to be introduced. As of 2017, LiFePO4 is a candidate for large-scale production of lithium-ion batteries, such as electric vehicle applications, due to its low cost, excellent safety, and high cycle durability. The energy density of an LFP battery is lower than that of other common lithium-ion battery types, such as Nickel Manganese Cobalt (NMC). Because of their lower cost, high safety, low toxicity, long cycle life, and other factors, LFP batteries are finding a number of roles in vehicle use, utility-scale stationary applications, and backup power. Working voltage = 3.0 ~ 3.3 V. Cycle life ranges from 2,700 to more than 10,000 cycles depending on conditions.
- Lithium Nickel Cobalt Aluminum Oxide (LiNiCoAlO2) – NCA. In 1999, Lithium nickel cobalt aluminum oxide battery, or NCA, appeared in some special applications, and it is similar to the NMC. It offers high specific energy, a long life span, and a reasonably good specific power. NCA’s usable charge storage capacity is about 180 to 200 mAh/g. The capacity of NCA is significantly higher than that of alternative materials such as LiCoO2 with 148 mAh/g, LiFePO4 with 165 mAh/g, and NMC 333 (LiNi0,33Mn0,33Co0,33O2)with 170 mAh/g. The voltage of these batteries is between 3.6 V and 4.0 V, at a nominal voltage of 3.6 V or 3.7 V. Another advantage of NCA is its excellent fast charging capability. Nevertheless, its weak points are the limited resources of cobalt and nickel and the high cost.
Chemistry of Electric Car Battery – How it works
An electric battery is essentially a source of DC electrical energy. It converts stored chemical energy into electrical energy through an electrochemical process. This then provides a source of electromotive force to enable currents to flow in electric and electronic circuits. A typical battery consists of one or more voltaic cells.
The fundamental principle in an electrochemical cell is spontaneous redox reactions in two electrodes separated by an electrolyte, which is an ionic conductive and electrically insulated substance.
But how does such a battery work?
In simple terms, each battery is designed to keep the cathode and anode separated to prevent a reaction. The stored electrons will only flow when the circuit is closed. This happens when the battery is placed in a device and the device is turned on.
When the circuit is closed, the stronger attraction for the electrons by the cathode (e.g. LiCoO2 in lithium-ion batteries) will pull the electrons from the anode (e.g. lithium-graphite) through the wire in the circuit to the cathode electrode. This battery chemical reaction, this flow of electrons through the wire, is electricity.
If we go into detail, batteries convert chemical energy directly to electrical energy. Chemical energy can be stored, for example, in Zn or Li, which are high-energy metals because they are not stabilized by d-electron bonding, unlike transition metals. Lithium metal is the lightest metal and possesses a high specific capacity (3.86 Ah/g) and an extremely low electrode potential (−3.04 V vs. standard hydrogen electrode). Therefore lithium is an ideal anode material for high-voltage and high-energy batteries.
During discharge, lithium is oxidized from Li to Li+ (0 to +1 oxidation state) in the lithium-graphite anode through the following reaction:
C6Li → 6C(graphite) + Li+ + e–
These lithium ions migrate through the electrolyte medium to the cathode, where they are incorporated into lithium cobalt oxide through the following reaction, which reduces cobalt from a +4 to a +3 oxidation state:
CoO2 (s) + Li+ + e– → LiCoO2 (s)
Here is the full reaction (left to right = discharging, right to left = charging):
C6Li + CoO2 ⇄ C6 + LiCoO2
These reactions can be run in reverse to recharge the cell. In this case, the lithium ions leave the lithium cobalt oxide cathode and migrate back to the anode, where they are reduced back to neutral lithium and reincorporated into the graphite network.
Batteries convert chemical energy directly to electrical energy. Chemical energy can be stored, for example, in Zn or Li, which are high-energy metals because they are not stabilized by d-electron bonding, unlike transition metals.
Even though many types of batteries exist with different combinations of materials, all of them use the same principle of the oxidation-reduction reaction. In an electrochemical cell, spontaneous redox reactions take place in two electrodes separated by an electrolyte, which is an ionic conductive and electrically insulated substance. The redox reaction is a chemical reaction that produces a change in the oxidation states of the atoms involved. Electrons are transferred from one element to another. As a result, the donor element, which is the anode, is oxidized (loses electrons), and the receiver element, the cathode, is reduced (gains electrons).
For example, the lithium-ion cell consists of two electrodes of dissimilar materials. The cathode is made of composite material and defines the name of the Li-ion battery cell. Cathode materials are generally constructed from LiCoO2 or LiMn2O4. Anode materials are traditionally constructed from graphite and other carbon materials. Graphite is the dominant material because of its low voltage and excellent performance. The electrolyte can be liquid, polymer (with a polymer gel as electrolyte), or solid. The separator is porous to enable the transport of lithium ions and prevents the cell from short-circuiting and thermal runaway.
During the charging process, Li+ ions move from the Li-containing anode and pass through the electrolyte-soaked separator, finally intercalating to the anode host structure. As a result, the electrons pass through the external circuit in the opposite direction.
During discharge, electrons flow through the external circuit through the negative electrode (anode) towards the positive electrode (cathode). The reactions during discharge lower the chemical potential of the cell, so discharging transfers energy from the cell to wherever the electric current dissipates its energy, mostly in the external circuit. During charging, these reactions and transports go in the opposite direction.
Degradation of Lithium-ion Batteries due to Cycling
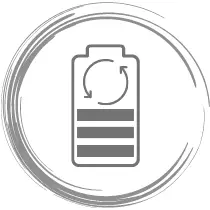
Some degradation of rechargeable batteries occurs on each charge-discharge cycle. Degradation usually occurs because electrolyte migrates away from the electrodes or because active material detaches from the electrodes. Manufacturers’ datasheet typically uses the word “cycle life” to specify lifespan in terms of the number of cycles to reach 80% of the rated battery capacity. Following the manufacturer’s recommendations is necessary to avoid danger or premature capacity degradation.
In lithium-ion batteries, degradation and capacity fade are generally attributed to the growth of the solid electrolyte interface (SEI). The solid electrolyte interface is created due to reactions between the electrodes and the electrolyte. These reactions form a film that hinders lithium ions from reacting with the electrodes, and as this film grows in thickness, the cell degrades.
In real-life applications, Li-ion cells experience accelerated degradation due to certain stress factors. Stress factors such as deep DODs, elevated C-rates, high or low temperatures, and operating at high SOCs can have a negative impact on the cell capacity and cause accelerated degradation.
- Temperature. Degradation is strongly temperature-dependent: degradation at room temperature is minimal but increases for batteries stored or used in hot or cold environments. Batteries generate heat when being charged or discharged, especially at high currents. High temperatures during charging may lead to battery degradation, and charging at temperatures above 45 °C will degrade battery performance. Large battery packs, such as those used in electric vehicles, are generally equipped with thermal management systems that maintain a temperature between 15 °C (59 °F) and 35 °C (95 °F).
- Elevated C-rate. High C-rates generate more heat and cause the temperature of the cell to rise invoking the high-temperature degradation mechanisms. C-rate reduces the usable life and capacity of a battery.
- DOD. For lithium-ion batteries, the cycle life of a cell strongly depends on the DOD. The loss of lithium ions and active electrode material is higher for larger DOD cycles. At high DODs, additional degradation mechanisms can occur, resulting in the decomposition and dissolution of cathode material and capacity fading.
Other Types of Batteries
The following list summarizes notable electric battery types composed of one or more electrochemical cells. Four lists are provided in the table. The first list is a battery classification by size and format. Then, the primary (non-rechargeable) and secondary (rechargeable) cell lists are lists of battery chemistry. The third list is a list of battery applications. The final list is a list of different battery voltages.
Sizes
- AA battery
- AAA battery
- AAAA battery
- C battery
- D battery
- cr1220 battery
- cr1620 battery
- cr1632 battery
- cr1616 battery
- cr2016 battery
- cr2032 battery
- cr2025 battery
- cr2430 battery
- cr2450 battery
- cr123 battery
- cr2 battery
- cr132a battery
- lr1130 battery
- lr41 battery
- lr44 battery
- A23 battery
- a13 battery
- 18650 battery
- 21700 battery