30-second summary
Permittivity
In electromagnetism, permittivity, which is also known as absolute permittivity, is a measure of the electric polarizability of a dielectric or an insulator.
A material with high permittivity, such as strontium titanate, polarizes more in response to an applied electric field than a material with low permittivity, thereby storing more energy in the material.
The relative permittivity, which is denoted by εr, is the same quantity as the dielectric constant denoted by κ (kappa).
The relative permittivity of a vacuum is unity by definition.
Vacuum permittivity, ε0, is approximately 8.85 x 10^-12 farads per meter (F/m) in SI units.
See also: Capacitance
See also: Electric polarization
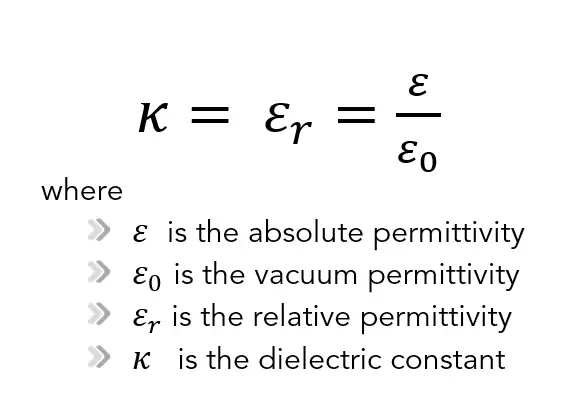
Permittivity – Absolute and Relative
In electromagnetism, permittivity, which is also known as absolute permittivity, is a measure of the electric polarizability of a dielectric or an insulator. The permittivity also characterizes the ability of the dielectric to store electric energy in an electrical field.
A material with high permittivity, such as strontium titanate, polarizes more in response to an applied electric field than a material with low permittivity, thereby storing more energy in the material. In electrostatics, permittivity plays an important role in determining the capacitance of a capacitor.
The absolute permittivity is often represented by the dimensionless relative permittivity, εr, which is the ratio of the absolute permittivity ε and the vacuum permittivity ε0.
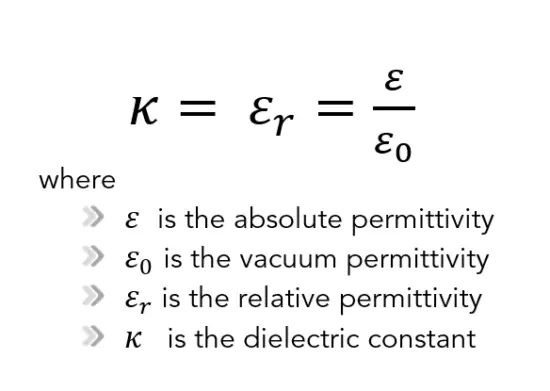
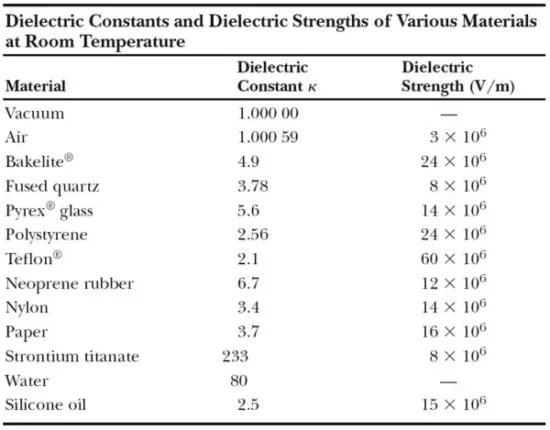
As can be seen, the main difference between relative and absolute permittivity is that relative permittivity is a dimensionless unit, while absolute permittivity units are farads per meter.
The relative permittivity, which is denoted by εr, is the same quantity as the dielectric constant denoted by κ (kappa). The dielectric constant is still used but deprecated by standards organizations in engineering.
The relative permittivity of a vacuum is unity by definition. Because air is mostly empty space, its measured relative permittivity is only slightly greater than unity (κair = 1.0006). Even common paper can significantly increase the capacitance of a capacitor, and some materials, such as strontium titanate, can increase the capacitance by more than two orders of magnitude.
The greater the relative permittivity, the greater the amount of charge that can be held. The capacitance of a capacitor is increased by a factor of relative permittivity when the gap between the plates is completely filled with a dielectric.
C = εrC0 = κeC0
where C0 is the capacitance between the plates with no dielectric.
Vacuum Permittivity
The vacuum permittivity (also called the permittivity of free space or the electric constant), ε0, is a physical constant that is a measure of how dense of an electric field is “permitted” to form in response to electric charges.
It is also connected to the energy stored within an electric field and capacitance. Perhaps more surprisingly, it’s fundamentally related to the speed of light. The vacuum permittivity is defined as:
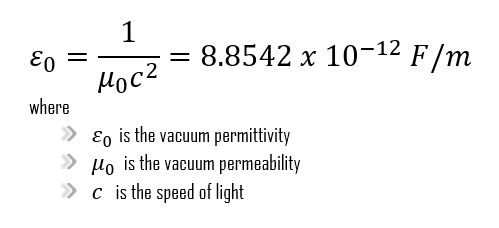
Electric Susceptibility
In electromagnetism, the electric susceptibility, χe, is a dimensionless proportionality constant that indicates the degree of polarization of a dielectric material in response to an applied electric field.
It is defined as the constant of proportionality of the induced dielectric polarisation density P to an electric field E.
If a dielectric material is a linear dielectric, then the ratio is:
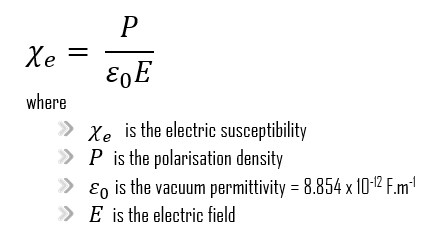
The susceptibility is proved to be related to its relative permittivity, also known as dielectric constant εᵣ by:
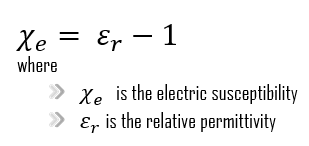
The greater the electric susceptibility, the greater the ability of a material to polarize in response to the field, thereby reducing the total electric field inside the material. It is in this way that the electric susceptibility influences the electric permittivity of the material and thus influences many other phenomena in that medium, from the capacitance of capacitors to the speed of light.
Electric Polarization
In contrast to metals, where charges are free to move throughout the material, in dielectrics, all the charges are attached to specific atoms and molecules. These charges are known as bound charges. These charges are able, however, can be displaced (polarized) within an atom or a molecule by an application of an electric field.
Electric polarization is a slight relative shift of positive and negative electric charge in opposite directions within atoms or molecules of an insulator, or dielectric, induced by an external electric field.
These microscopic displacements are not as dramatic as the rearrangement of charge in a conductor, but their cumulative effects account for the characteristic behavior of dielectric materials. When an external electric field is applied to a dielectric material, this material becomes polarized, which means that it acquires a dipole moment. This property of dielectrics is known as polarizability. When an electric field acts on a molecule, the positive charges are displaced along the field, while the negative charges are displaced in a direction opposite to that of the field. The effect is, therefore, to pull the opposite charges apart, i.e., to polarize the molecule. There are three types of polarization:
- Electronic polarization. Here when the external field is applied, the electron clouds of atoms are displaced with respect to the heavy nuclei within the dimensions of these atoms. This is called electronic polarization.
- Orientational polarization. Orientational polarization is a polarization that is either inherent to molecules, or can be induced in any molecule in which the asymmetric distortion of the nuclei is possible (distortion polarization). Polar molecules are those types of dielectrics in which the chances of positive and negative molecules colliding are nil or zero. This is because they are all asymmetrical in form. H2O is a typical example. When there is no electric field, the electric dipole moment of these molecules moves in an unpredictable direction. The average dipole moment is 0 as a result of this. If there is an external electric field, the molecules will assemble in the same direction as the electric field.
- Ionic polarization. Ionic polarization is polarization caused by relative displacements between positive and negative ions in ionic crystals (for example, NaCl).
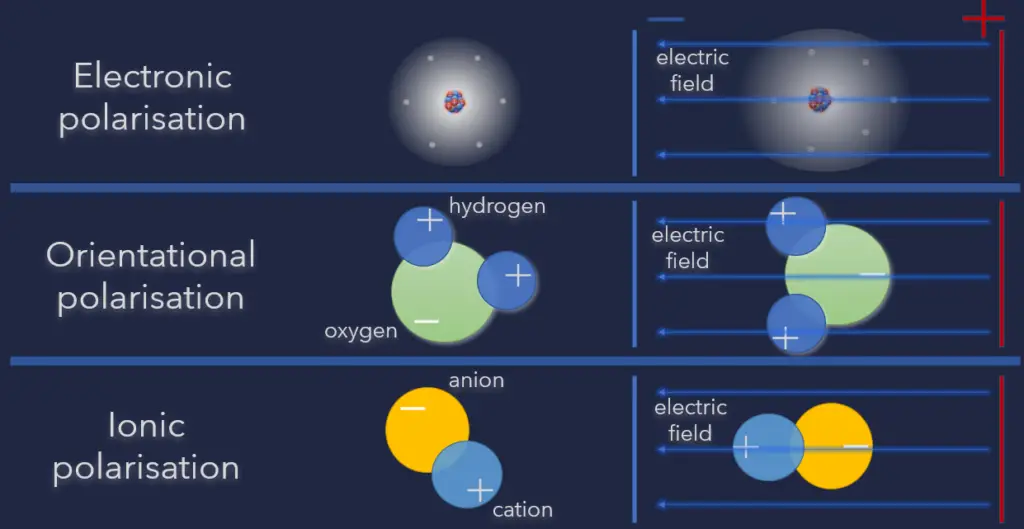
Dielectrics in Capacitors
Dielectrics have many applications, but the most significant use is in capacitors. In many capacitors, there is an insulating material such as paper or plastic between the plates. Such a material, called a dielectric, can be used to maintain a physical separation of the plates.
Placing a solid dielectric between the plates of a capacitor serves three functions.
- Mechanical separation
- Electrical isolation – higher voltage possible
- Electric field reduction – higher capacitance
First, it solves the mechanical problem of maintaining two large metal sheets at a very small separation without actual contact.
Second, using a dielectric increases the maximum possible potential difference between the capacitor plates. Any insulating material, when subjected to a sufficiently large electric field, experiences a partial ionization that permits conduction through it. This is called dielectric breakdown. Note that the dielectric strength of air is approximately 3 kV/mm. Many dielectric materials can tolerate stronger electric fields without breakdown than can air. Thus using a dielectric allows a capacitor to sustain a higher potential difference and so store greater amounts of charge and energy.
Third, experimentally, it was found that capacitance C increases when the space between the conductors is filled with dielectrics. The polarization of the dielectric by the applied electric field increases the capacitor’s surface charge for the given electric field strength. The application of an electric field between the two plates induces some opposite charge on the dielectric, which opposes the applied electric field. The net result is that the electric field inside the dielectric (which fills the area between the plates) is reduced, allowing the capacitor to store more charge.
To see how this happens, suppose a capacitor has a capacitance when there is no material between the plates. When a dielectric material is inserted to completely fill the space between the plates, the capacitance increases to:
C = κeC0
where κe is called the dielectric constant.
As can be seen, there are two cases. If the potential difference between the plates of a capacitor is maintained, as by battery B, the effect of a dielectric is to increase the charge on the plates. (b) If the charge on the capacitor plates is maintained, as in this case, the effect of a dielectric is to reduce the potential difference between the plates.
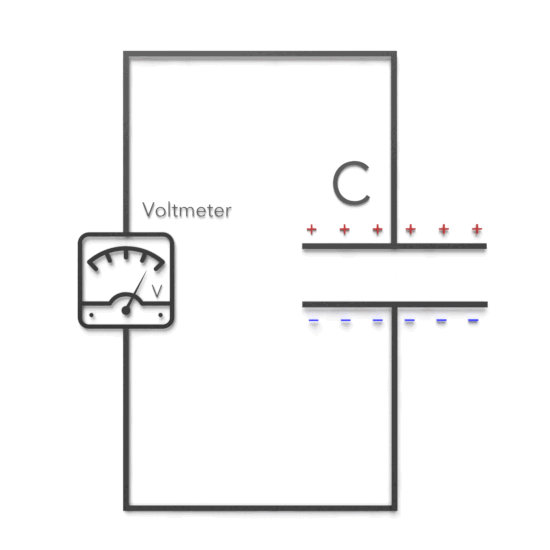
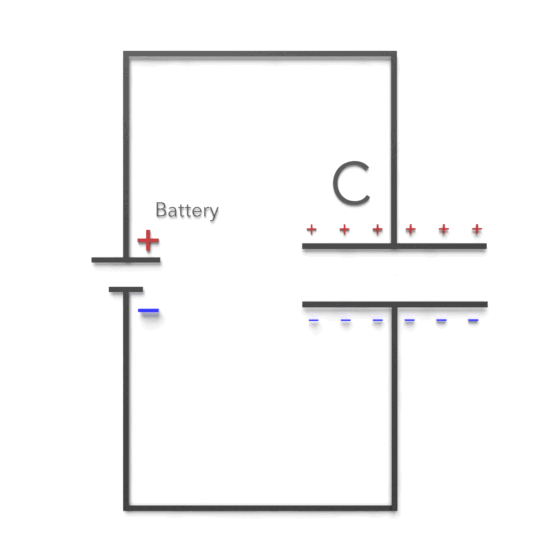